Phylogenetic Patterns in Mouth Posture and Echolocation Emission Behavior of Phyllostomid Bats
- 1Institute of Evolutionary Ecology and Conservation Genomics, Ulm University, Ulm, Germany
- 2Smithsonian Tropical Research Institute, Panamá City, Panamá
- 3Department of Biology, University of Southern Denmark, Odense, Denmark
While phyllostomid bats show an impressive range of feeding habits, most of them emit highly similar echolocation calls. Due to the presence of an often prominent noseleaf, it has long been assumed that all phyllostomids emit echolocation calls exclusively through the nostrils rather than through the mouth. However, photo evidence documents also phyllostomid bats flying with an opened mouth. We hypothesized that all phyllostomid species emit echolocation calls only through the nostrils and therefore fly consistently with a closed mouth, and that observations of an open mouth should be a rare and random behavior among individuals and species. Using a high-speed camera and standardized conditions in a flight cage, we screened 40 phyllostomid species. Behavior varied distinctly among the species and mouth posture shows a significant phylogenetic signal. Bats of the frugivorous subfamilies Rhinophyllinae and Carolliinae, the nectarivorous subfamilies Glossophaginae and Lonchophyllinae, and the sanguivorous subfamily Desmodontinae all flew consistently with open mouths. So did the animalivorous subfamilies Glyphonycterinae, Micronycterinae and Phyllostominae, with the notable exception of species in the omnivorous genus Phyllostomus, which consistently flew with mouths closed. Bats from the frugivorous subfamily Stenodermatinae also flew exclusively with closed mouths with the single exception of the genus Sturnira, which is the sister clade to all other stenodermatine species. Further, head position angles differed significantly between bats echolocating with their mouth closed and those echolocating with their mouths opened, with closed-mouth phyllostomids pointing only the nostrils in the direction of flight and open-mouth phyllostomids pointing both the nostrils and mouth gape in the direction of flight. Ancestral trait reconstruction showed that the open mouth mode is the ancestral state within the Phyllostomidae. Based on the observed behavioral differences, we suggest that phyllostomid bats are not all nasal emitters as previously thought and discuss possible reasons. Further experiments, such as selectively obstructing sound emission through nostrils or mouth, respectively, will be necessary to clarify the actual source, plasticity and ecological relevance of sound emission of phyllostomid bats flying with their mouths open.
Introduction
The majority of bats depend on echolocation for both orientation and foraging. Echolocation is a highly complex behavior and can be adjusted to the specific ecological needs of a species in many ways (Denzinger et al., 2018). Several different echolocation call designs have evolved that vary widely in temporal and spectral parameters, call intensity, and beam width (e.g., Heller and Helversen, 1989; Brinkløv et al., 2009; Surlykke et al., 2013; Jung et al., 2014).
Echolocation calls can be emitted orally or nasally (Metzner and Müller, 2016). Families considered to be oral emitters are, e.g., Emballonuridae, Mormoopidae, Noctilionidae, Molossidae, and most Vespertilionidae (Pedersen, 1993). Individuals from these groups emit calls from the mouth, keeping the mouth widely open during flight, as seen in photographs of flying individuals, e.g., Noctilio leporinus (Noctilionidae), Eumops glaucinus (Molossidae, Taylor and Tuttle, 2019), and Hypsugo bodenheimeri (Vespertilionidae, Kounitsky et al., 2015). On the other hand, bats in several other families are known to echolocate through the nostrils, e.g., Rhinolophidae and Hipposideridae (Metzner and Müller, 2016). These species usually keep their mouths closed during flight (e.g., Rhinolophus hildebrandtii, Hipposideros ruber; Taylor and Tuttle, 2019). In addition, they often have prominent structures surrounding their nostrils, including so-called noseleaves (e.g., Vanderelst et al., 2013; Webala et al., 2019; Wilson and Mittermeier, 2019). Both Hipposideridae and Rhinolophidae – two families with often very elaborate noseleaves – are exclusively insectivorous and hunt in narrow space habitats (Bell and Fenton, 1984; Neuweiler et al., 1987; Bontadina et al., 2002; Denzinger and Schnitzler, 2013). To be able to differentiate prey from the background they use glint detection and narrow space flutter detection, respectively (reviewed by Schnitzler and Denzinger, 2011). A third family with very prominent noseleaves are the Phyllostomidae (Arita, 1990; Bogdanowicz et al., 1997; Leiser-Miller and Santana, 2020). This exclusively Neotropical family includes currently 217 species (Wilson and Mittermeier, 2019) and is highly diverse in foraging behavior, diet and habitat use (Ferrarezzi and Gimenez, 1996; Kalko et al., 1996; Giannini and Kalko, 2004; Surlykke et al., 2014).
Similar to Hipposideridae and Rhinolophidae, many phyllostomid bats are narrow space foragers that hunt in dense forest (Denzinger et al., 2018). In contrast to the mainly insectivorous hipposiderids and rhinolophids, phyllostomid bats feed on a huge variety of resources with diet specificity roughly reflected by the systematic classification into subfamilies. Animalivorous species feed mostly on insects, but some include small vertebrates (e.g., Micronycterinae, Glyphonycterinae, and most Phyllostominae, Giannini and Kalko, 2004). Other phyllostomids are mostly phytophagous, predominantly consuming fruits or pollen and nectar (e.g., Stenodermatinae, Carolliinae, Glossophaginae, Lonchophyllinae, Giannini and Kalko, 2004). Furthermore, there are three phyllostomid species that feed exclusively on the blood of vertebrates (Desmodontinae, Fenton, 1992).
Generally, echolocation is shaped by the respective ecological niche (Schnitzler and Kalko, 2001; Jones and Holderied, 2007), so we expect differences between species with differing foraging behaviors. However, on first glance most phyllostomid bat species use very similar echolocation calls, which are usually short (<2 ms), multiharmonic, frequency-modulated (FM), and cover a broad bandwidth (Kalko, 2004; Korine and Kalko, 2005; Weinbeer and Kalko, 2007; Gessinger, 2016; Yoh et al., 2020). The free-standing noseleaves found in almost all phyllostomid bats are thought to play an essential role in the emission of these echolocation calls (Hartley and Suthers, 1987; Arita, 1990; Vanderelst et al., 2010). Reduced noseleaves are only found in the two subfamilies Desmodontinae and Brachyphyllinae (Wilson and Mittermeier, 2019). Because of the ubiquitous noseleaves, phyllostomid bats are generally considered to be nasal emitters (Hartley and Suthers, 1987; Matsuta et al., 2013; Jakobsen et al., 2018; Brokaw and Smotherman, 2020). Additionally, anatomical features of the skull indicate that phyllostomid bat echolocation is optimized for emission through the nostrils. Phyllostomids have straight air flow from the larynx to the nostrils, whereas sound emitted through the mouth has to travel a longer and less direct path (Pedersen, 1993, 1998).
Phyllostomid bats in flight can be seen in many photographs with their mouths closed, as would be expected for nasal emitters, e.g., Mesophylla macconnelli, Phyllostomus discolor (López-Baucells et al., 2016). However, a closer inspection of own and published photographs revealed also bats flying with a partially opened mouth, e.g., Trachops cirrhosus, Lophostoma silvicolum, Carollia perspicillata, and Desmodus rotundus (Figure 1), Carollia castanea (Taylor and Tuttle, 2019), Hsunycteris (Lonchophylla) thomasi, Artibeus gnomus, Phylloderma stenops, Lophostoma carrikeri, and Micronycteris microtis (López-Baucells et al., 2016). This behavior has also been commented on by Fenton (2013).
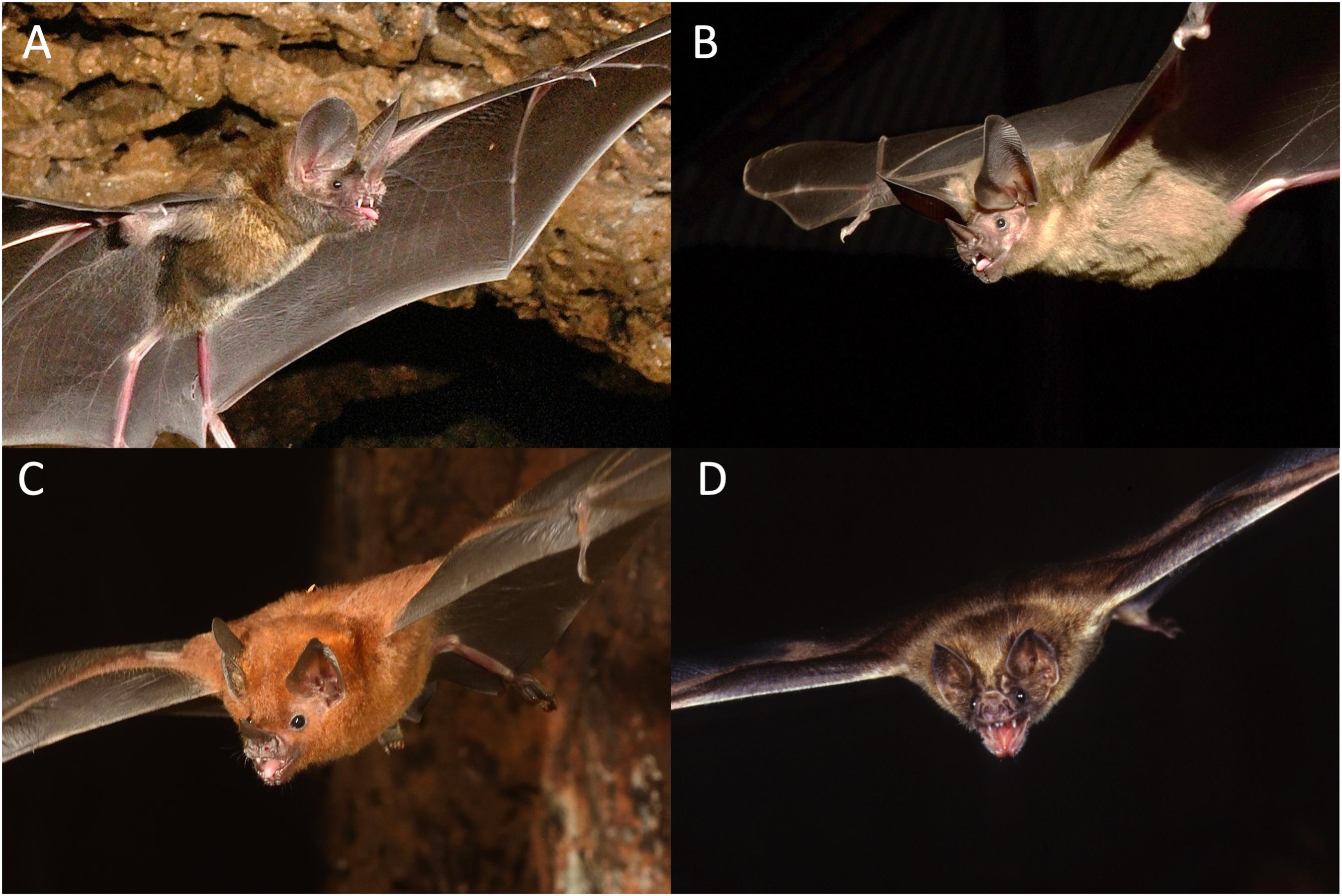
Figure 1. Phyllostomid bats photographed in flight with open mouths. (A) Trachops cirrhosus, (B) Lophostoma silvicolum, (C) Carollia perspicillata, (D) Desmodus rotundus (Photos by MT).
We therefore asked whether these open-mouth observations reflect only random and occasional behavior of individuals, or whether specific behavioral patterns exist among the phyllostomid species that can be related to the emission mode of echolocation calls.
Specifically, we focused on the following hypotheses, based on the general view that the noseleaf is an adaptation for nasal echolocation:
1. Phyllostomid bat species with free-standing noseleaves are consistent nasal emitters and fly with the mouth closed.
2. The head position enables the emitter (nostrils) to point in the main direction of flight.
To investigate these hypotheses, we recorded high-speed video footage of the behavior of 40 phyllostomid species in a flight cage under standardized conditions and quantitatively assessed the head position and degree of mouth opening (measured as gape angle).
Materials and Methods
Study Sites
Bats were captured at five different Neotropical field sites over a five-year period (2015–2019) in Panamá and Perú: 1. Field station Barro Colorado Island (BCI) of the Smithsonian Tropical Research Institute (STRI) and the surrounding Barro Colorado Nature Monument in Panamá (9° 9′ 0′′ N, 79° 51′ 0′′ W) between March and July 2015. 2. Field station Gamboa of the Smithsonian Tropical Research Institute and nearby Soberanía National Park, Panamá (9°07′11.5′′N 79°42′18.2′′W), June to July 2016, and April 2019. 3. Las Pavas, Panamá (9°05′21.1′′N 79°52′37.5′′W), July 2015 and June 2016. 4. Sachavacayoc Center at the river Rio Tambopata, south-east of Puerto Maldonado, Madre de Dios, Perú (12°51′11.9 S, 69°22′2.3 W), September 2017. 5. Allpahuayo-Mishana National Reserve, Iquitos, Perú (3°56′39.0′′S 73°36′23.4′′W), August 2018. Individuals in Panamá were partly captured for the study of Brändel et al. (2020). Individuals from Peru were partly captured during a bat course organized in 2018 by CEBIO (Centro de Biodiversidad).
Studied Bats
Phyllostomid bats of 40 species were captured with mistnets. Individuals were from both sexes and belonged to ten subfamilies: Micronycterinae, Desmodontinae, Phyllostominae, Glossophaginae, Lonchorhininae, Lonchophyllinae, Glyphonycterinae, Carollinae, Rhinophyllinae, and Stenodermatinae (Rojas et al., 2016). We used the phylogeny of Noctilionoidea by Rojas et al. (2016). To identify species, we used specific identification literature for Panamá (Handley et al., unpublished) and Perú (Díaz et al., 2011; López-Baucells et al., 2016; Reid, unpublished), respectively. A characteristic feature of the phyllostomid bats is a fleshy appendage on the nose, the so-called noseleaf (Wilson and Mittermeier, 2019). Most species within the family, including our study species have a free-standing noseleaf that is mostly taller than wide. The subfamily with the consistently smallest noseleaves is the subfamily Desmodontinae (or vampire bats), where the nasal structures are reduced to low folds or ridges. Following Wilson and Mittermeier (2019) we therefore classified Desmodus rotundus as the only species in our data set with a reduced noseleaf.
High Speed Videos
In order to score whether the bats flew with their mouths open or closed, we hand-released each individual following a standardized protocol in a flight cage (Panamá, permanent outdoor flight cage, approximately 360 × 250 × 230 cm (length × width × height); Perú, EUREKA screen house (hexagon), approximately 395 × 420 × 235 cm (length × width × height) while filming with a high-speed video camera (Optronis CR600 × 2, 8 GB memory) at resolutions between 1,280 × 800 pixels and 1,280 × 1,024 pixels. The camera was placed at a distance of ca. 40 cm from the releaser at a 90° angle to the anticipated, ideal flight path of the bats on a parallel line at ca. 30 cm to the right (Supplementary Figure 1). For most species we used a 20 mm lens (Sigma, 1:1.8) at an aperture of 4–5.6, which covers approximately 40 cm of the flight path. For small species we occasionally used a 50 mm lens (Nikkor: 1:1.2) at an aperture of 4–5.6, which covered approximately 20 cm of the flight path. We recorded at 500 frames per second at an exposure time of 1/2,000 or 1/3,000 s, using a pre- and post-trigger period of 2 s each. The recordings were triggered manually with a handheld trigger as soon as the bat started flying. Bats were illuminated with infrared spotlights (Panamá: Uniflood 500 Strahler, 230 V, 220 W, 730 nm; Perú: AEGIS UFLED Intelligent-IR Strahler 20-8BD, BOSCH, 230 V, 45 W, 850 nm). To verify echolocating behavior, we monitored the ultrasound range (Avisoft Bioacoustics UltraSoundGate 416H; microphone: Avisoft-Bioacoustics, CM16, sampling rates 300 and 500 kHz) and found that bats were consistently echolocating during all flight sequences. After the recording session, we released the bats at the site of capture, usually within the same night.
We obtained 331 sequences of 177 individuals of 40 species to determine whether the mouth was open or closed during flight. From these, we selected 1 to 8 representative videos for each species for further measurements. We used one recording per individual, selecting the recording with the straightest flight path and best video quality, for measurements of gape angle (degree of mouth opening) and head position angle on 2 to 5 representative frames. Occasionally, individuals exhibited both conditions – mouth opened and closed – in consecutive flights. In this case we selected one video from each mode, resulting in two videos of this individual. For measuring gape angle, we drew two axes following the upper (palate) and lower (dentary) jawbone and measured the angle in between (Supplementary Figure 2A). Additionally, to measure the head position angle, we drew a line along the main body axis and crossed it with a second line drawn centered between the upper and lower jawbone (Supplementary Figure 2B). We used the angle tool of the software ImageJ (http://rsb.info.nih.gov/ij), measured every angle three times (digitalization error 2° per 3 measurements, Supplementary Table 2) and calculated the mean value. Hereafter, we refer to bats flying with the mouth open (gape angle > 0°) as “open-mouth bats” and those flying with the mouth closed as “closed-mouth bats”.
Roost Emergence Photographs
For Lonchorhina aurita, in addition to flight cage observations described above, we also took photographs during roost emergence in Soberanía National Park, Panamá. We used a Nikon D810 with a 20–70 mm zoom lens with an open shutter, while the flash (Nikon SB900, set to 1/8) was triggered by the emerging bat interrupting a light beam. We obtained 75 photographs in which the mouth was clearly visible. Whenever teeth were visible, the individual was scored as an “open-mouth bat,” otherwise as a “closed-mouth bat.”
Phylogenetic Analysis
To test for a phylogenetic signal in the mouth posture trait, we constructed a phylogeny of the species included in this study based on the sequences provided in Rojas et al. (2016). In total, we were able to include 39 taxa; the Genbank IDs of sequences are indicated in Supplementary Table 3. The subspecies of L. silvicolum used in this study (L. silvicolum silvicolum) was not included in Rojas et al. (2016), we therefore used the genetically well-characterized L. silivicolum laephotis. For the newly described Sturnira giannae, we used species-specific sequences (pers. comm. P. Velazco, Supplementary Table 3). We had to exclude Artibeus ravus because sequences are unavailable for this species.
We used Beast 1.10.4 to reconstruct a phylogenetic tree. The available sequence data consist of up to 9 nuclear and mitochondrial sequence fragments, including both coding and non-coding sequences; we curtailed fragments so they either only contained coding or non-coding sequences. We used the SRD06 model of sequence evolution (Shapiro et al., 2006) as a nucleotide substitution model for the coding sequences. For the non-coding sequences, we used the same parameters (substitution model: HKY, estimated base frequencies, gamma-distributed site heterogeneity with 4 gamma categories), but without partitioning the sequence into codon positions. For the substitution model, we linked the priors in four groups: nuclear coding fragments (atp7, bdnf, ttn6, rag2); nuclear non-coding fragments (plcb4, stat5a, thy); mitochondrial coding fragments (cox1, cytb) and mitochondrial non-coding fragments (12s). We used the relaxed lognormal clock as a clock model, linking priors into two groups (nuclear and mitochondrial) without time calibration. We used the flexible GMRF skyride as a demographic tree prior (Minin et al., 2008), linking priors across all fragments. All models were run long enough to obtain effective sample sizes >200 for all parameters with a 10% burn-in, and checked for convergence in Tracer (v1.7.1). We used TreeAnnotator v.1.10.4 to produce Maximum Clade Credibility (MCC) trees. To reconstruct the ancestral states of mouth posture, we performed a discrete trait analysis in Beast 1.10.4 with an asymmetric substitution model for mouth posture. The tree was visualized in FigTree v.1.4.4.
We then used the MCC tree generated in Beast (without the discrete trait) and the fitDiscrete function in the geiger package in R to calculate Pagel’s lambda. We generated a null model (no phylogentic signal, lambda = 0) and a model assuming Brownian motion evolution (lambda = 1) and compared models with a log-likelihood test.
Statistical Analysis
We used R (version 2.6.2, 2008-02-08) for all statistical analyses. To compare the two groups, ‘open-mouth emitters’ and ‘nasal emitters’ we used the Wilcoxon rank sum test. As we found a significant phylogenetic signal for mouth posture, we additionally performed a phylogenetic analysis using the phylANOVA function in the phytools package in R to test whether mouth posture (three states: open, closed and “both”) significantly affects mean head position angle; the drop.tip function was used to remove the tip for Glossophaga soricina, for which no head angle data was available. For species in which both open and closed mouth occurred, we used an exact binominal test to determine which was the more common behavior. All statistical analyses were performed using a significance level of α = 0.05.
Results
Mouth Posture
We obtained 331 flight sequences from 177 individuals of 40 phyllostomid bat species (Table 1, Figure 2, and Supplementary Video 1). Sixteen species consistently flew with the mouth closed. Five species exhibited both closed and open mouths in flight. Nineteen species consistently flew with the mouth open. These patterns were not distributed randomly across the phylogenetic tree; rather they reflect the phylogenetic relationships of these species as shown by reconstructing ancestral traits with a discrete trait analysis in Beast 1.10.4 (Figure 3). An open mouth posture was reconstructed as the ancestral state of the Phyllostomidae. The closed mouth posture has evolved at least in two independent clades, within the Stenodermatinae and the genus Phyllostomus. Mouth posture shows a significant phylogenetic signal (Pagel’s lambda λ = 0.799, log-likelihood-test, p < 0.001); λ did not significantly differ from Brownian motion evolution (p = 0.13).
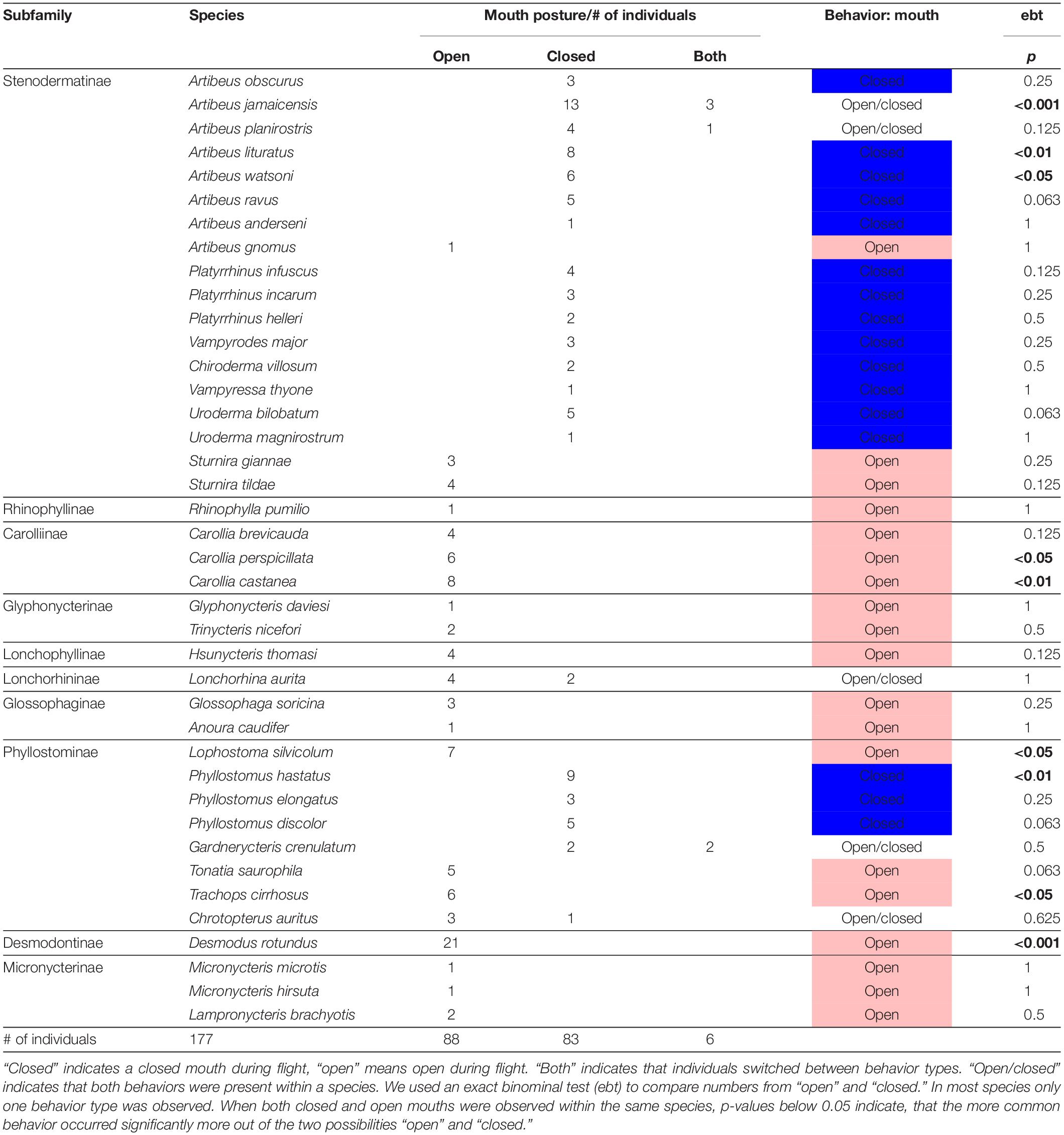
Table 1. Scoring of the mouth posture of 40 phyllostomid species during flight (mean number of sequences scored per individual: 2).
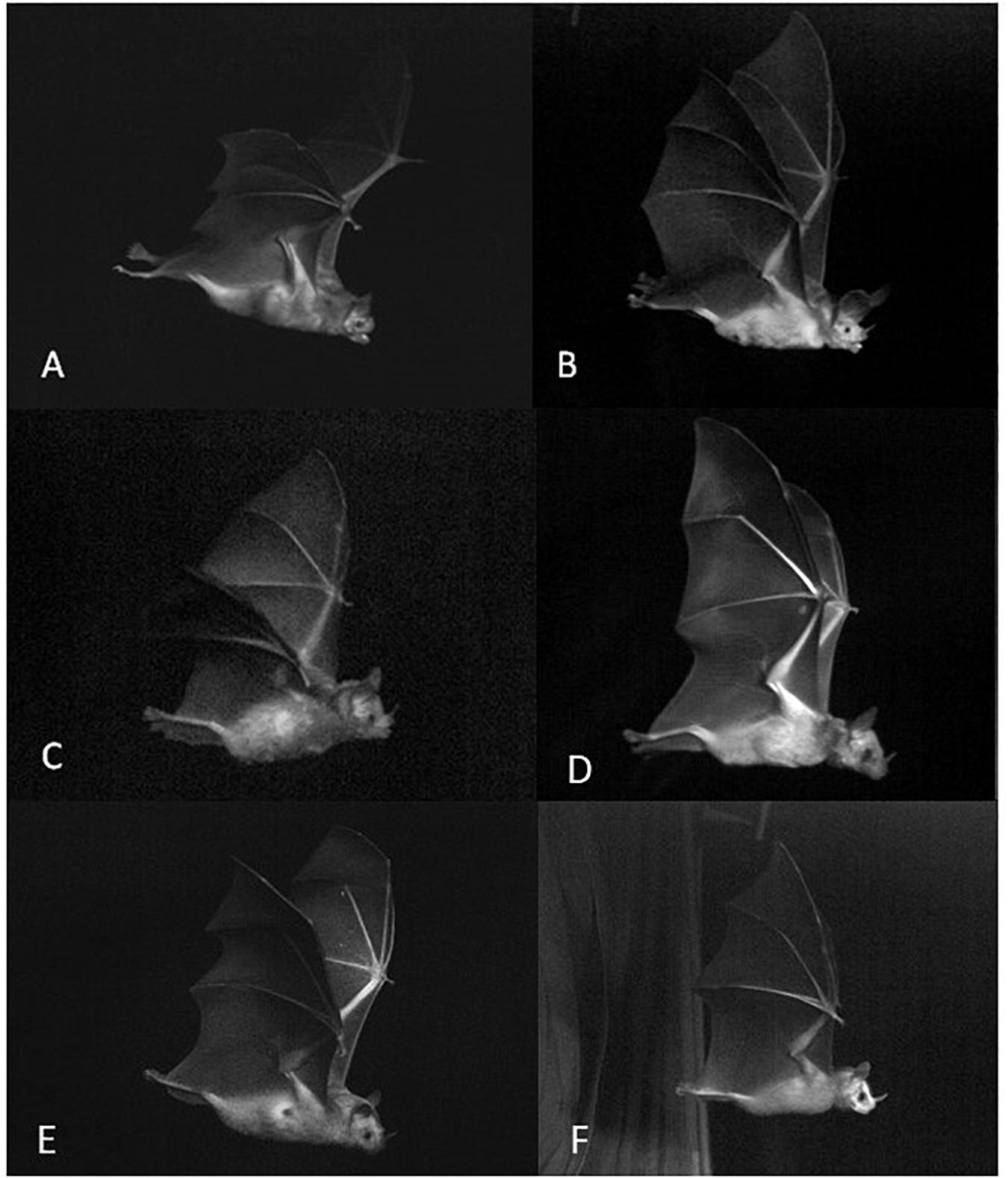
Figure 2. Examples of still shots extracted from the high-speed videos. Open-mouth phyllostomids (gape angle > 0°): (A) Desmodus rotundus, (B) Lophostoma silvicolum, (C) Carollia castanea. Nasal emitters: (D) Phyllostomus discolor, (E) Artibeus jamaicensis, (F) Vampyrodes major.
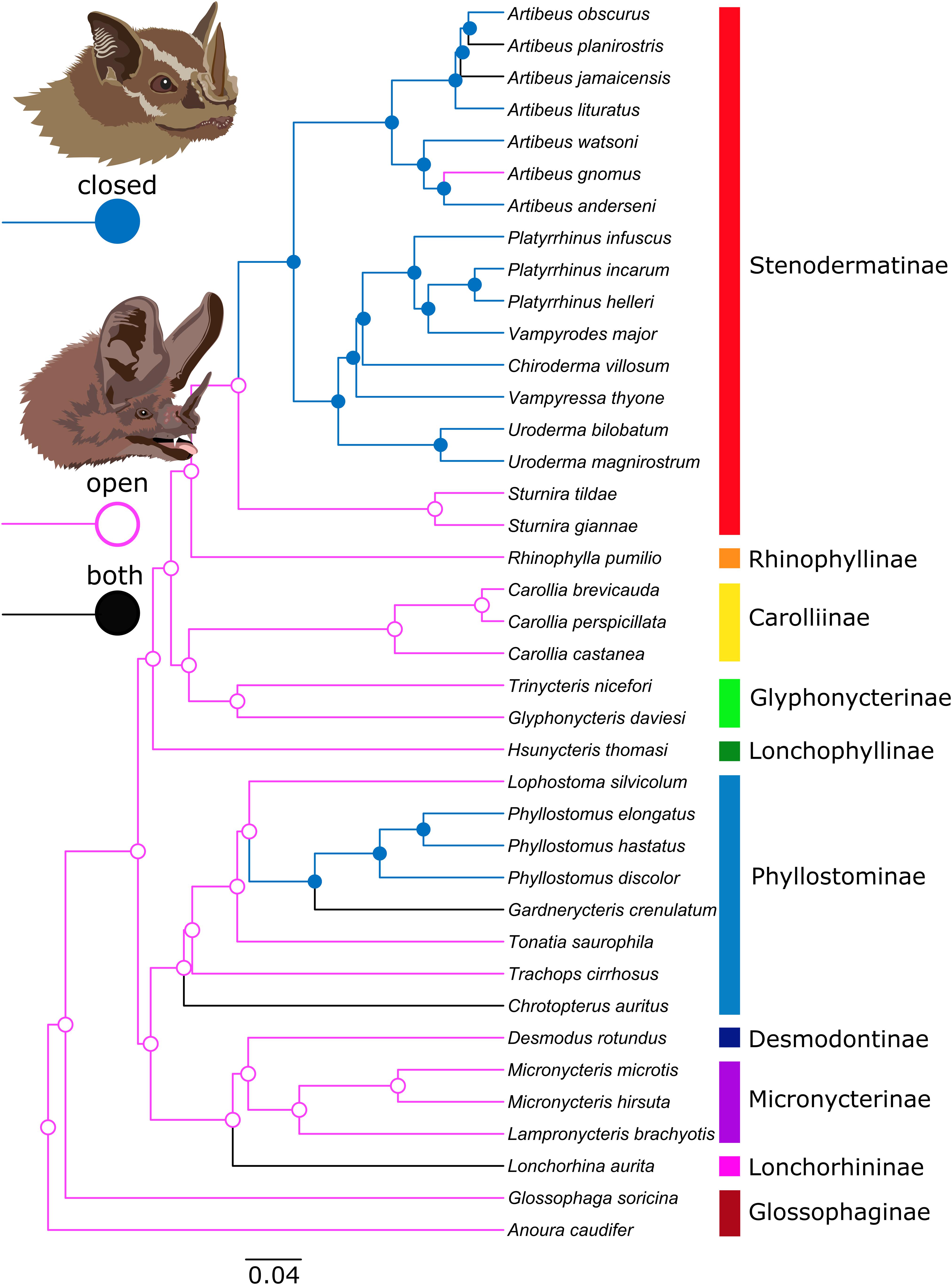
Figure 3. Ancestral state reconstruction of mouth posture. The MCC tree was constructed with TreeAnnotator v.1.10.4 on Beast v1.10.4 runs using sequence data provided in Rojas et al. (2016) (see Supplementary Table 3). Mouth posture was reconstructed as a discrete trait with an asymmetric substitution rate. Colors and node shapes indicate the mouth posture state (pink/light circle = open-mouth species; blue/filled circle = closed-mouth species; black = “both”). Posterior support is shown on the tree. Subfamilies are indicated by rainbow colors (Illustrations by D. Kyllo).
Most bats from the Stenodermatinae, except two species of the genus Sturnira, consistently flew with the mouth closed and we therefore consider them closed-mouth species. Additionally, all three scored species of the genus Phyllostomus (Phyllostominae) were closed-mouth species (Table 1).
Within a species most individuals showed the same behavior and maintained the mouth either consistently open or consistently closed (Table 1). Inconsistent behavior occurred within the Stenodermatinae, Lonchorhininae and Phyllostominae. In two species, the behavior varied across individuals. Of the six individual Lonchorhina aurita recorded in the flight cage, four individuals flew with mouths consistently open, while two flew with mouths consistently closed. Similarly, of the four Chrotopterus auritus recorded, three flew with the mouth consistently open, while one individual always flew with the mouth closed. Six individuals (3.4%) from three species out of our entire sample (n = 177 individuals) switched between open-mouth and closed-mouth mode in consecutive flights. Of the 16 individual Artibeus jamaicensis recorded, three individuals flew with the mouth slightly open in one out of two sequences. Two of these three had the mouth closed in the first sequence and open in a second sequence. One individual had the mouth open in the first sequence, closed in the second, and reopened in the third sequence. In addition, one of the five Artibeus planirostris individuals recorded had the mouth opened in the first out of three sequences. Individuals of Gardnerycteris crenulatum showed the greatest behavioral variation. One of the four individuals used open-mouth mode in the first and third trial, and closed-mouth mode during the second and fourth trial. A second individual kept the mouth open during the first flight and closed during the next four flights. The last two individuals were only recorded using closed-mouth mode (in one and two flights, respectively).
Desmodus rotundus (Desmodontinae), the only species in our data set with a reduced noseleaf, consistently flew with an opened mouth. All species of the subfamilies Micronycterinae, Glossophaginae, Carolliinae, Lonchophyllinae, and Glyphonycterinae were also consistently open-mouth species. Additionally, certain species within both Phyllostominae (Lophostoma silvicolum, Tonatia saurophila, and Trachops cirrhosus) and Stenodermatinae (Artibeus gnomus, Sturnira tildae, and Sturnira giannae) were open-mouth species (Table 1).
Gape Angle
Among the open-mouth species, gape angle varied from 19° in Artibeus gnomus to 54° in Desmodus rotundus (Figure 4, Table 2).
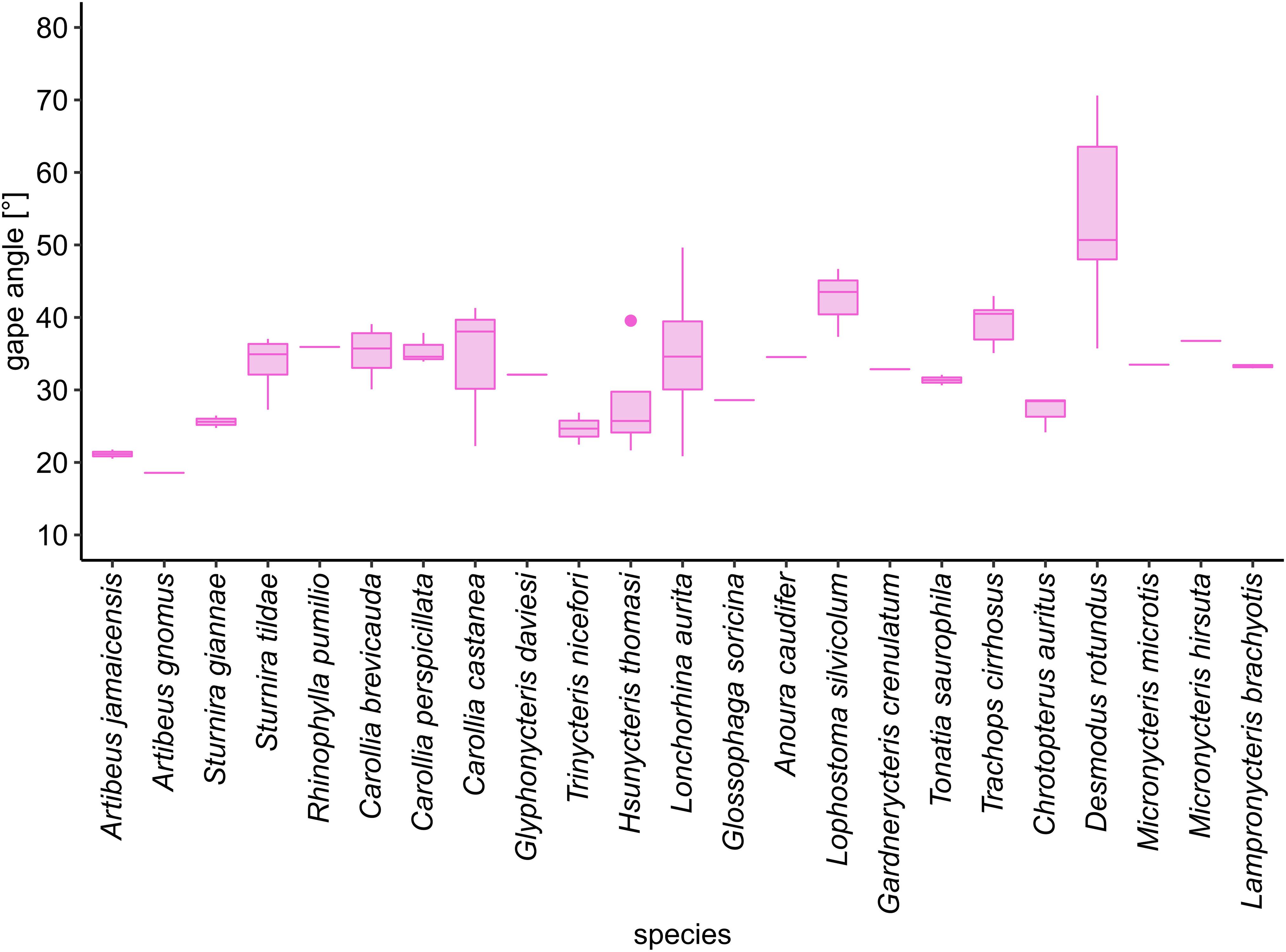
Figure 4. Gape angle of 23 phyllostomid species. Desmodus rotundus had the widest gape angle (54° ± 12.1°) while Artibeus gnomus (19°) opened the mouth only slightly during flight. The box incorporates the middle 50% of the data and the line within the box the median value. Whiskers mark the 25th percentile and the 75th percentile of the data (range of the data). Outliers are plotted as dots (values that are more than 1.5 times the interquartile range beyond either end of the box).
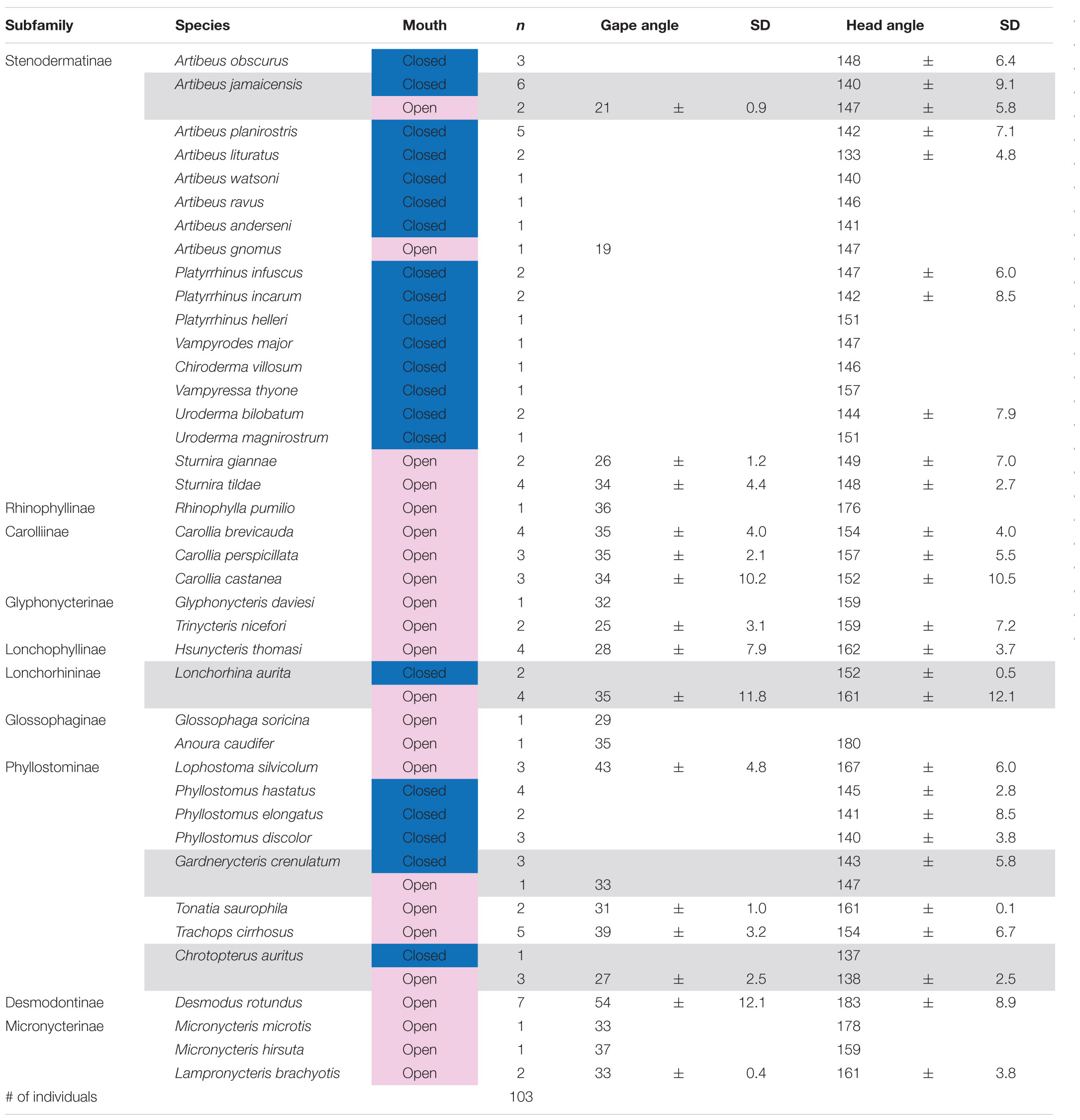
Table 2. Mean gape angle in degree [°] of 23 species and head position angle of 40 species of phyllostomid bats. Nasal emitters had their mouth consistently closed and no gape angle could be measured. Gray background indicates that individuals of this species were observed with open and closed mouth. For Glossophaga soricina no head position angle could be measured. n is the number of individuals. SD is the standard deviation.
Head Position Angle
Open-mouth species and closed-mouth species differed significantly in their head position angle (Wilcoxon rank sum test, W = 51, p < 0.001) (Figure 5). This difference in head angle according to mouth posture was confirmed by a phylogenetic ANOVA, including open, closed and “both” as mouth posture states (F = 16.377, p < 0.01). A post hoc test shows that head position significantly differs both between species with open and closed mouths (t = 3.771, p < 0.05) and with open and ambiguous (“both”) mouth posture (t = 5.272, p < 0.05), but not between closed and ambiguous states (t = 0.122, p = 0.916). Open-mouth species held their heads straighter, resulting in a larger angle (mean = 159° ± 11.8 SD). Desmodus rotundus even bent its head slightly upwards (mean = 183° ± 8.9 SD). Closed-mouth species had a smaller head position angle, indicating they tilted their heads more downwards (mean = 144° ± 5.4 SD). We found the smallest angle of head position for Artibeus lituratus at 133°, followed by a closed-mouth individual of Chrotopterus auritus at 137°. Even within species, individuals flying with an open mouth held the head straighter than individuals flying with a closed mouth (Table 2).
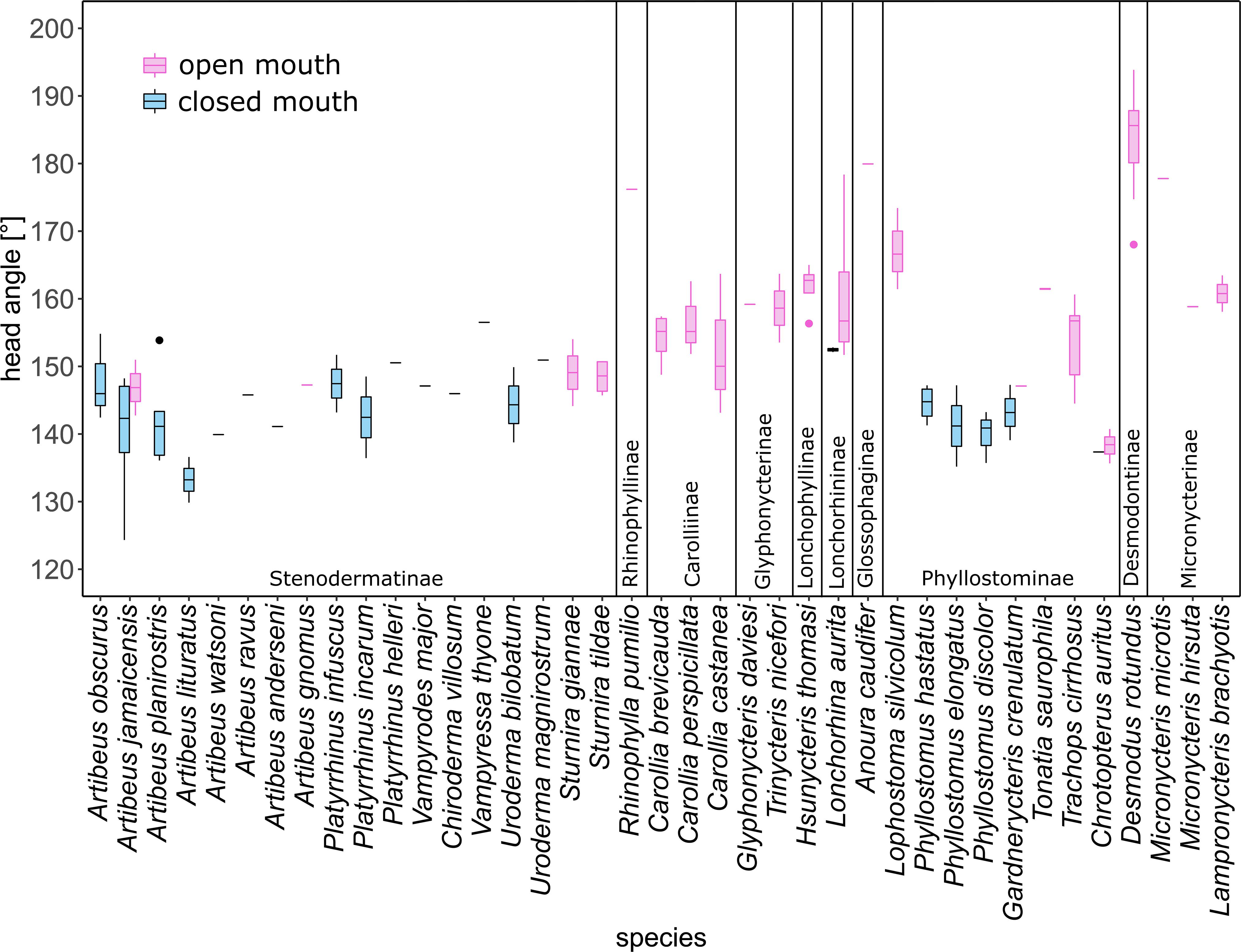
Figure 5. Boxplots of mean head position angle per species. Vertical lines separate subfamilies. Open-mouth species held the head straighter (mean = 159° ± 12) than individuals with closed mouths (nasal emitters) (mean = 183° ± 8). The box incorporates the middle 50% of the data and the line within the box the median value. Whiskers mark the 25th percentile and the 75th percentile of the data (range of the data). Outliers (values more than 1.5 times the interquartile range beyond either end of the box) are plotted as dots.
Roost Emergence Photographs
In addition to the flight cage observations of Lonchorhina aurita (n = 6 individuals), we scored 75 photographs of L. aurita emerging from their roost in the evening. In 41 photographs the mouth was closed (no teeth showing), while in 34 photographs the mouth was open (teeth visible) (Supplementary Figure 3). There was no significant difference in number of observations between these two behaviors (exact binominal test, probability of closed mouth: 0.55, p = 0.49).
Discussion
Phyllostomidae is one of the ecologically most diverse families of bats. Yet, phyllostomid species show remarkably homogenous echolocation call structure, often assumed to be associated with the presence of a noseleaf, and, by extension, nasal emission of calls. In the context of recent growing interest in phyllostomid echolocation behavior (Gonzalez-Terrazas et al., 2016; Rodríguez-San Pedro and Allendes, 2017; Gessinger et al., 2019; Yoh et al., 2020; Zamora-Gutierrez et al., 2020), we explored mouth and head angle postures during echolocation within this family to make predictions about call emission modes. Following anecdotal and photographic evidence of phyllostomid bats flying with an open mouth, we investigated whether this behavior occurs occasionally or consistently across the family. We used high speed video recordings of a wide range of phyllostomid species in flight to show that the majority (88%) of the sampled species flew either consistently with the mouth opened or consistently with the mouth closed. Every bat in our study echolocated during the recordings. Supported by the consistent differences of the head position angle, we suggest that our observations of closed- and open-mouth species, reflect the emission mode of echolocation calls. Further, distribution of the closed- and open-mouth mode among the species was not random but showed distinct phylogenetic patterns supported by Pagel’s lambda statistic. While bats flying with a closed mouth can emit echolocation calls only through the nostrils (nasal emission), open-mouth species may use: i) nasal emission, ii) oral emission, iii) alternating nasal and oral emission, iv) synchronous nasal and oral emission. The context in which plastic emitters shift between emission modes, and the possible ecological advantages of one emission mode over another in a given context, present future avenues of study.
With few exceptions, mouth posture (open/closed) and head angle were remarkably consistent among the individuals of a species. While the sample sizes are heterogeneously distributed among the species, our study across 40 phyllostomid species indicates a phylogenetic pattern in phyllostomid echolocation behavior. Below, we first show the distribution of the observed behaviors over the phylogenetic tree of the phyllostomid family (Figure 3) and then discuss their implications for echolocation.
Phylogenetic Patterns
We hypothesized that phyllostomid bats with free-standing noseleaves would be exclusively nasal emitters and not, or only occasionally show an open mouth. However, we found that only 16 out of 40 species (40.0%) always flew with the mouth closed. In contrast, we found that 19 species (47.5%) consistently kept the mouth open during flight (Table 1 and Figure 3). While we believe that this is representative behavior in most of our study species, we are less confident of this pattern in species with low sample sizes. The behavior of the single individual of Artibeus gnomus, for example, might have occurred by chance, because it contrasts with the main pattern found for the entire genus Artibeus. In only five species (12.5%), we found both behaviors to occur, which in some species might reflect natural variability that is better detected in larger sample sizes, such as in Artibeus jamaicensis, where 13 out of 16 animals (81%) always maintained a closed mouth.
Closed-Mouth Mode
In support of our first hypothesis, 16 species with free-standing noseleaves used closed-mouth behavior, never showed an open mouth and thus were confirmed to be nasal emitters.
Within the Stenodermatinae subfamily, our sampling of the genera Platyrrhinus, Vampyrodes, Chiroderma, Vampyressa, and Uroderma revealed only nasal emitters. This group is the sister clade to the clade containing all species from the genus Artibeus (Figure 3), from which all individuals included in our study also maintained a closed mouth, confirming them to be nasal emitters. Only few individuals of A. jamaicensis and A. planirostris switched in consecutive flights from open-mouth to closed-mouth mode. This might indicate that they used both behaviors. Alternatively, the open mouth in these individuals could be unrelated to echolocation, but be an indicator, e.g., of stress related to handling. No individual of A. jamaicensis was observed flying exclusively with an open mouth and with 13 individuals flying exclusively with a closed mouth we reached the statistical power to confirm the original hypothesis of nasal emission (Table 1). In A. planirostris we observed also no individuals exclusively flying with an open mouth and a higher sampling effort would show whether nasal emission is indeed the most used behavior. The only member of the genus Artibeus we scored flying exclusively with an open mouth was A. gnomus. Unfortunately, we captured only a single individual of this species and obtained only a single recording that we could analyze. It would be important to explore whether the open mouth is really the consistent mode in this species or if this behavior changes between trials or individuals. In summary, for all eight Artibeus species tested, 40 out of 45 individuals or 89% used nasal emission exclusively.
Within the Phyllostominae subfamily, all scored individuals from the three species of the genus Phyllostomus were exclusively observed with a closed mouth. While for Phyllostomus hastatus sampling effort was high enough for a significant result, we did not have the sufficient sample sizes to conclude the same for P. discolor and P. elongatus (Table 1).
Besides these, some individuals from Gardnerycteris crenulatum, Chrotopterus auritus, and Lonchorhina aurita – had their mouths entirely closed at least occasionally, indicating exclusive nasal emission in these situations. In the case of G. crenulatum, two individuals started to fly with an open mouth but continued flying with a closed mouth in consecutive trials. An open mouth at the beginning of recordings in a nasal-emitting species could also be a sign, e.g., of stress as mentioned above. Two other individuals had the mouth consistently closed. Therefore, while nasal emission in this species is possible, our results are inconclusive. The same is true for C. auritus; three individuals flew with open mouth and teeth visible, another exclusively with a closed mouth. We cannot rule out that our experimental setup, adapted primarily for small to medium sized bats, affected the behavior of C. auritus, the largest species sampled in our data set. To clarify whether G. crenulatum and C. auritus use both open-mouth and closed-mouth mode in equal percentages, a larger sample size and a setup adjusted for large body size would be helpful. In contrast, L. aurita (Lonchorhininae) clearly used both behaviors; four individuals consistently flew with an open mouth (67%) and two with a closed mouth (33%), and neither behavior was significantly preferred (binomial test, p = 1). Additional roost emergence photographs further indicate equal use of both behaviors, with approximately half of the individuals flying with their mouth open (45%) and half with their moth closed (55%). L. aurita has the longest noseleaf of the Phyllostomidae, so an open mouth contradicts our original hypothesis. However, L. aurita stands out in this family by using long echolocation calls with constant frequency components (Gessinger et al., 2019). The inconsistent behavior of L. aurita might therefore actually reflect a sensory adaptation (see below).
Open Mouth Mode
The common vampire bat Desmodus rotundus (Desmodontinae), the only species in our data set with a reduced noseleaf (Wilson and Mittermeier, 2019), is an open-mouth species. However, we also found that all sampled species from the subfamilies Micronycterinae, Glossophaginae, Rhinophyllinae, Carolliinae, Lonchophyllinae, and Glyphonycterinae, all with free-standing noseleaves, are open-mouth species. This contradicts our first hypothesis that species with free-standing noseleaves are inherently nasal emitters. An interesting exception within the Stenodermatinae is the genus Sturnira, where both tested species (S. giannae and S. tildae) showed also only open-mouth individuals.
Summarizing our results, echolocation emission behaviors of phyllostomid bats are not randomly distributed over the phylogenetic tree (Figure 3) and mouth posture shows a significant phylogenetic signal. Ancestral trait reconstruction showed that the open mouth mode is the ancestral state within the Phyllostomidae and that exclusive nasal emission (closed mouth) evolved at least twice in two independent clades (Stenodermatinae and the genus Phyllostomus). We suggest that exclusive nasal emission evolved within the large subfamily Stenodermatinae. While the genus Sturnira, which is the sister clade to all other Stenodermatinae, uses the open-mouth mode, almost all other Stenodermatinae, comprising 45% of the sampled species, are exclusive nasal emitters. Within the subfamily Phyllostominae, exclusive nasal emission evolved at least once in the genus Phyllostomus. Phyllostomus hastatus, P. discolor and P. elongatus, representing three of the four extant species of the genus, showed exclusively nasal emission and were never observed with an open mouth. Additionally, exclusive nasal emission may have evolved at least twice more, in the genera Gardnerycteris and Chrotopterus, although this is unresolved as both behavior types were observed in these species.
In conclusion, as a whole phyllostomids are not characterized by a single behavior type. Rather, open-mouth or closed-mouth behavior is dominant or exclusively used within most phyllostomid subfamilies, which we posit has implications for the echolocation emission mode of this ecologically diverse group of bats. Higher behavioral variability is only found within the Phyllostominae, which happens to also be the ecologically most variable family, including on one hand animalivorous gleaners and “carnivores” but also omnivores feeding on insects, fruits and nectar (Gardner, 1977; Hoffmann et al., 2008). Open-mouth mode is reconstructed to be the ancestral behavior (Figure 3).
Echolocation Call Emission
Echolocation calls of laryngeally echolocating bats are either emitted orally, nasally, or in a combined manner through three sources, the two nostrils and the mouth (Jakobsen et al., 2018). In most families this trait seems to be remarkably constant (Metzner and Müller, 2016).
Closed-Mouth Species
Anatomical features of the skull (Pedersen, 1995, 1998) and the presence of an often prominent noseleaf (Arita, 1990; Bogdanowicz et al., 1997) have been seen as evidence for nasal emission of echolocation calls in the Phyllostomidae (Hartley and Suthers, 1987; Matsuta et al., 2013; Jakobsen et al., 2018; Brokaw and Smotherman, 2020). Bats keeping their mouth completely closed must emit echolocation signals exclusively through the nostrils (e.g., Rübsamen, 1987; Suthers et al., 1988). We confirm that this is indeed the case for 16 phyllostomid species, all of which feed mainly on plant products (Giannini and Kalko, 2004).
Small sound-emitting structures such as the nostrils of a bat produce a much broader beam than larger structures, e.g., its mouth, for any given frequency (Kounitsky et al., 2015). Many phyllostomid bats forage in dense forest where a broader beam is unfavorable because it will result in more distracting echoes reflected by the background vegetation (Kalko et al., 2008; Denzinger et al., 2018). Generally, the beam can be narrowed either by enlarging the emitter size or by using higher frequencies (Jakobsen et al., 2013; Kounitsky et al., 2015). Bat nostrils as separate entities have a rather fixed size and – unlike mouth gapes – can probably not be enlarged enough to significantly narrow the beam width (Brokaw and Smotherman, 2020). However, a signal emitted by more than one emitter (e.g., two nostrils), may experience interference phenomena that can narrow the beam in the horizontal plane. Blocking one nostril in Carollia perspicillata resulted in horizontal widening of the beam pattern (Hartley and Suthers, 1987). Similar, an emission without the lancet of the noseleaf was shown to widen the emission pattern in the vertical plane in Carollia perspicillata (Hartley and Suthers, 1987), and in a model of Phyllostomus discolor and Micronycteris microtis (Vanderelst et al., 2010). Finally, in the nasally emitting rhinolophid Rhinolophus ferrumequinum beam width modeled without noseleaf was larger than the beam width actually measured, also suggesting that the noseleaf focuses the beam (Strother and Mogus, 1970).
Open-Mouth Species
Nineteen species in our study consistently flew with an open mouth. Mouth opening could be involved in shaping the echolocation beam during flight. As mentioned above, open-mouth bats have four different options for the emission of echolocation calls: 1. Nasally, through the nostrils, 2. Through the mouth, 3. Alternately through mouth and nostrils, 4. Synchronously through mouth and nostrils.
Nasally
Emission of echolocation calls through nostrils integrated into a noseleaf has the advantage of a reduced vertical and horizontal beam width (Hartley and Suthers, 1987; Vanderelst et al., 2010). Phyllostomid bats usually filter out the fundamental harmonic and use several higher harmonics instead (Yoh et al., 2020). This is probably achieved through a filtering process in the nasal cavities, as demonstrated for Rhinolophus hildebrandti (Rhinolophidae). By sealing the nostrils bats were forced to echolocate through the mouth, which lead to a sudden increase of the energy in the fundamental harmonic, probably because the filtering got disturbed (Suthers et al., 1988). Therefore, opening the mouth could even be disadvantageous for phyllostomids. Perching Trachops cirrhosus have been observed to occasionally echolocate with an open mouth, which resulted in some animals in an increase of intensity of the first harmonic (Surlykke et al., 2013). Anatomical evidence for an exclusively nasal sound emission can be found in the Hipposideridae and Rhinolophidae. In those bat families the lid of the larynx fits perfectly into the nasolaryngeal opening, thus creating a clear separation between the mouth- and the larynx-to-nostril air flow (Neuweiler, 1993). To our best knowledge, such a separation has not been discovered for phyllostomids, however, information on the anatomy of the vocal tract of phyllostomid species seems to be scarce.
Orally
Bats emitting echolocation calls orally can change the beam width by adjusting the mouth gape. Mouth-emitting Bodenheimer’s pipistrelle bats Hypsugo bodenheimeri (Vespertilionidae) achieve a narrower beam width by increasing their mouth gape when flying into cluttered environments (Kounitsky et al., 2015). This easy way to adjust beam width could be used by open-mouth phyllostomids in their narrow space forest habitats. In Desmodus rotundus, with its almost entirely reduced noseleaf, we actually scored a large variability in mouth gape, suggesting that this species might adjust the mouth gape and therefore also beam width during flight. All other open-mouth phyllostomids in our study had free-standing noseleaves and we consider it unlikely that these structures would have evolved if echolocation calls were emitted exclusively orally.
Alternating oral and nasal emission
A bidirectional echolocation mode involving both nasal and oral emission has been suggested in Barbastella barbastellus (Vespertilionidae). This aerial hunting bat emits two alternating echolocation call types, one of which is presumably emitted through the nostrils and the other through the mouth. In a single channel recording this alternation can be detected by distinct shifts in intensity, as the two sound beams differ in their orientation toward the microphone (Seibert et al., 2015). It is suggested that the nasally emitted calls serve mainly for detection and localization of prey, while the orally emitted call supports spatial orientation.
Simultaneous nasal and oral emission
Emitting sound through a nostril causes a large beam width due to the small emitter size. Beam width can be horizontally decreased through interference of the two nostril sound beams and vertically controlled by the noseleaf (Hartley and Suthers, 1987; Vanderelst et al., 2010). Also, vertical beam could be narrowed by adding a third point source, i.e., oral sound emission. The measured sound beam of the vespertilionid Plecotus auritus was best explained by a triple-emitter model involving both nostrils and the mouth. This model was the only one consistent with the observed narrowing of the beam in the vertical plane (Jakobsen et al., 2018). So far, directionality of echolocation calls of open-mouth phyllostomids has only been studied for Carollia perspicillata and Trachops cirrhosus (Brinkløv et al., 2011; Surlykke et al., 2013). Both species showed narrow sonar beam widths. Interestingly, beam width of Carollia perspicillata was narrower than in previous studies where oral emission was prevented by sealing the mouth (Hartley and Suthers, 1987), supporting the idea of synchronous mouth and nostril emission.
Since open-mouth mode is the ancestral behavior in the phyllostomid tree the question arises, why stenodermatines and the species of the genus Phyllostomus do not use the open-mouth mode? Perhaps their generally rather broad noseleaves are specially adapted to focusing the beam in the vertical plane, thus making additional oral emission unnecessary. On the other hand the advantages of being able to carry relatively large fruits might outweigh the advantages of a more narrow echolocation beam, especially as they may also use olfactory cues during foraging (Korine and Kalko, 2005; Hodgkison et al., 2013; Ripperger et al., 2019). A narrow beam could be more important for animalivorous gleaning bats (e.g., Trachops cirrhosus and Lophostoma silvicolum), which listen to prey- generated sounds, have to capture potentially mobile prey and face a dilemma between calling and listening (Jones et al., 2016). A narrow beam produces less irrelevant echoes potentially interfering with the sounds produced by the prey. In this context, a future comparison of beam widths between nasal emitters and open-mouth species might be extremely interesting.
Several species pointed their nostrils in the direction of flight, confirming our second hypothesis that head position enables the emitters to point in the main direction of flight. Open mouth individuals showed a larger head position angle, pointing both mouth gape and nostrils in the flight direction. Even within a species, individuals flying with an open mouth held their head straighter than individuals flying with closed mouths. However, due to the small sample size we could not obtain more conclusive data. Nevertheless, the difference in head position between the two behavioral groups further supports the idea that call emission through the mouth is much more prominent than previously thought in phyllostomid echolocation. The combined evidence of mouth posture and head angle therefore suggests that open-mouth phyllostomids use a synchronous emission of echolocation calls through mouth and nostrils.
Open-Mouth Mode/Nasal Emission
While everything mentioned above for open-mouth species and closed-mouth species applies for species using both open-mouth mode and closed-mouth mode as well, the question arises why a few species sometimes fly with an open mouth and sometimes keep their mouth closed. The clearest case here is Lonchorhina aurita, where a solid sample size underlines an almost equal use of nasal emission and open-mouth mode. Lonchorhina aurita shows an echolocation call design strikingly different from all other phyllostomid genera, composed of a constant frequency (CF) and a frequency modulated (FM) component (Gessinger et al., 2019). One explanation would be that the two components could be emitted separately through nostrils and mouth, respectively. Synchronized high-speed video and audio recordings would be necessary to test this hypothesis and, combined with recent advances in bat tagging technology, might shed light on the functional significance of this pattern (Stidsholt et al., 2021).
Conclusion
With the 40 species included in our study we assembled a phylogenetically broad sample that covers almost all subfamilies and close to 20% of extant species of the ecologically highly diverse phyllostomid family. While sample size in some species is admittedly limited, our study reveals for the first time an intriguing phylogenetic pattern within the family. Within almost all phyllostomid subfamilies we found a remarkably uniform mouth posture and matching head position. An interesting exception were the Phyllostominae, where the genus Phyllostomus showed the same closed-mouth posture (indicating nasal emission) as did the vast majority of the exclusively plant-visiting species from the Stenodermatinae subfamily. This coincides with the fact that the genus Phyllostomus is ecologically distinct from other, exclusively animalivorous species in the phyllostomines, by being highly omnivorous, consuming many insects and even small vertebrates like the other phyllostomines, but utilizing also a high proportion of plant resources (fruit, nectar). These results suggest that diet and the corresponding foraging behavior might influence the actual emission type. In conclusion, the considerable proportion of open-mouth species and the significant difference of head position angles between open-mouth species and nasal emitters highly suggests that the phyllostomid family contains not just exclusively nasal-emitting species. Future investigation may reveal additional fully or partly oral-emitting species to be mapped on the phylogenetic phyllostomid tree. Experiments, such as selectively obstructing sound emission through nostrils or mouth, respectively, will be necessary to clarify to what extent open-mouth phyllostomids are emitting sound through the mouth and/or nostrils, respectively, and the potential consequences for echolocation beam width and niche adaptation in the ecologically highly diverse phyllostomid family.
Data Availability Statement
The datasets presented in this study can be found in the article/Supplementary Material under the link: https://www.frontiersin.org/articles/10.3389/fevo.2021.630481/full#supplementary-material.
Ethics Statement
The animal study was reviewed and approved by STRI’s animal ethics committee (IACUC protocols 2014-0101-2017 and 2017-0102-2020). We followed the guidelines for the use of wild mammal species in research as recommended by the American Society of Mammalogists.
Author Contributions
GG, AS, and MT had the idea for the study. GG and MT made the flight cage recordings in Panamá and Perú. GG did the video analysis, prepared figures and tables, and drafted the manuscript. LW did the phylogenetic analysis. GG and LW did the statistics. GG, RP, SB, LW, and MT contributed to finishing the manuscript. All authors gave final approval for publication. All authors contributed to the article and approved the submitted version.
Funding
GG received a DAAD – PROMOS – grant and a Smithsonian Fellowship for conducting fieldwork. GG was funded by the Heinrich-Böll-Stiftung. Additional funds were provided by the University of Ulm, Germany.
Conflict of Interest
The reviewer, SS, declared a past co-authorship with one of the authors, MT, to the handling editor.
The remaining authors declare that the research was conducted in the absence of any commercial or financial relationships that could be construed as a potential conflict of interest.
Acknowledgments
We thank the Batlabs on BCI and in Gamboa and the entire Smithsonian Tropical Research Institute in Panamá for their great support. We especially want to mention Stefan Brändel and Thomas Hiller for sharing captured bats, Tania Witt for support in genetic species identification, as well as Gregg Cohen for invaluable help with logistics and administration, and Inga Geipel for scientific encouragement. In Perú we are grateful for the kind support from Erika Paliza, CEBIO Perú, and want to thank also all participants and teachers of the “Curso de Murcielagos (2018).” Further we would like to thank Paul Velazco for support in species identification and for clarification on sequence data for Sturnira giannae, and David Pascall for discussions on phylogenetic analysis. We are also grateful to David Brugers and the entire staff at Sachavacayoc Center for their excellent support. The comments of Brock Fenton, Liliana Davalos, Sharlene Santana, and two further referees helped to significantly improve the manuscript.
Supplementary Material
The Supplementary Material for this article can be found online at: https://www.frontiersin.org/articles/10.3389/fevo.2021.630481/full#supplementary-material
Supplementary Figure 1 | Setup for high speed video recordings: bat illustration indicating the point of release, blue error indicating the anticipated, ideal flight path of the bat, infrared lights (IR) and high-speed cagmera (camera) located at ca. 30 cm at a 90° angle on a parallel line at ca. 40 cm distance to point of release.
Supplementary Figure 2 | Schematic drawings illustrating the measured angles: (A) two axes were drawn along the upper (palate) and lower (dentary) jawbone and the gape angle measured in between; (B) a line along the main body axis was drawn and crossed with a second line drawn between the upper and lower jawbone (angle dissector of the gape angle) and the head position angle was measured.
Supplementary Figure 3 | Examples of Lonchorhina aurita photographed during roost emergence. In 41 individuals no teeth were visible and their mouth posture was scored as closed (left). 34 individuals were scored with open mouths because teeth were clearly visible (right) (Photos by MT).
Supplementary Table 1 | Raw data of i. mouth posture, ii. gape and head position angle, iii. mouth posture of L. aurita during roost emergence.
Supplementary Table 2 | Angle measurements and their digitalization errors.
Supplementary Table 3 | Genbank sequence IDs of the sequences used for reconstructing the phylogenetic tree, modified from Rojas et al. (2016) and pers comm. Paul Velazco.
Supplementary Video 1 | High speed videos of flying Phyllostomid bat, slowed down by factor 10. Species: Artibeus jamaicensis, Plathyrrinus incarum, Vampyrodes major, Uroderma magnirostrum, Carollia brevicauda, Carollia perspicillata, Carollia castanea, Hsunycteris thomasi, Phyllostomus hastatus, Tonatia saurophila, Trachops cirrhosus, and Lampronycteris brachyotis.
References
Arita, H. T. (1990). Noseleaf morphology and ecological correlates in phyllostomid bats. J. Mammal. 71, 36–47. doi: 10.2307/1381314
Bell, G. P., and Fenton, M. B. (1984). The use of Doppler-shifted echoes as a flutter detection and clutter rejection system: the echolocation and feeding behavior of Hipposideros ruber (Chiroptera: Hipposideridae). Behav. Ecol. Sociobiol. 15, 109–114. doi: 10.1007/BF00299377
Bogdanowicz, W., Csada, R. D., and Fenton, M. B. (1997). Structure of noseleaf, echolocation, and foraging behavior in the Phyllostomidae (Chiroptera). J. Mammal. 78, 942–953. doi: 10.2307/1382954
Bontadina, F., Schofield, H., and Naef-Daenzer, B. (2002). Radio-tracking reveals that lesser horseshoe bats (Rhinolophus hipposideros) forage in woodland. J. Zool. 258, 281–290. doi: 10.1017/S0952836902001401
Brändel, S. D., Hiller, T., Halczok, T. K., Kerth, G., Page, R. A., and Tschapka, M. (2020). Consequences of fragmentation for Neotropical bats: the importance of the matrix. Biol. Conserv. 252:108792. doi: 10.1016/j.biocon.2020.108792
Brinkløv, S., Jakobsen, L., Ratcliffe, J. M., Kalko, E. K. V., and Surlykke, A. (2011). Echolocation call intensity and directionality in flying short-tailed fruit bats, Carollia perspicillata (Phyllostomidae)a). J. Acoust. Soc. Am. 129, 427–435. doi: 10.1121/1.3519396
Brinkløv, S., Kalko, E. K. V., and Surlykke, A. (2009). Intense echolocation calls from two “whispering” bats, Artibeus jamaicensis and Macrophyllum macrophyllum (Phyllostomidae). J. Exp. Biol. 212, 11–20. doi: 10.1242/jeb.023226
Brokaw, A. F., and Smotherman, M. (2020). Role of ecology in shaping external nasal morphology in bats and implications for olfactory tracking. PLoS One 15:e0226689. doi: 10.1371/journal.pone.0226689
Denzinger, A., and Schnitzler, H.-U. (2013). Bat guilds, a concept to classify the highly diverse foraging and echolocation behaviors of microchiropteran bats. Front. Physiol. 4:164. doi: 10.3389/fphys.2013.00164
Denzinger, A., Tschapka, M., and Schnitzler, H.-U. (2018). The role of echolocation strategies for niche differentiation in bats. Can. J. Zool. 96, 171–181. doi: 10.1139/cjz-2017-0161
Díaz, M. M., Aguirre, L. F., and Barquez, R. M. (2011). Clave de Identificación de los Murciélagos del Cono Sur de Sudamérica. Cochabamba: Centro de Estudios en Biología Teórica y Aplicada.
Fenton, M. B. (1992). Wounds and the origin of blood-feeding in bats. Biol. J. Linn. Soc. 47, 161–171. doi: 10.1111/j.1095-8312.1992.tb00662.x
Fenton, M. B. (2013). Questions, ideas and tools: lessons from bat echolocation. Anim. Behav. Incl. Special Sec. Behav. Plast. Evol. 85, 869–879. doi: 10.1016/j.anbehav.2013.02.024
Ferrarezzi, H., and Gimenez, E. D. A. (1996). Systematic patterns and the evolution of feeding habits in Chiroptera (Archonta: Mammalia). J. Comp. Biol. 1, 75–94.
Gardner, A. L. (1977). Feeding Habits. Biology of the New World Family Phyllostomidae. Lubbock: H. Texas Tech Press.
Gessinger, G. (2016). Oral Emission of Echolocation Calls in Phyllostomid Bats? Master’s thesis. Germany: Ulm University.
Gessinger, G., Gonzalez-Terrazas Tania, P., Page Rachel, A., Kirsten, J., and Marco, T. (2019). Unusual echolocation behaviour of the common sword-nosed bat Lonchorhina aurita: an adaptation to aerial insectivory in a phyllostomid bat? R. Soc. Open Sci. 6:182165. doi: 10.1098/rsos.182165
Giannini, N. P., and Kalko, E. K. V. (2004). Trophic structure in a large assemblage of phyllostomid bats in Panama. OIKOS 105, 209–220. doi: 10.1111/j.0030-1299.2004.12690.x
Gonzalez-Terrazas, T. P., Koblitz, J. C., Fleming, T. H., Medellín, R. A., Kalko, E. K. V., Schnitzler, H.-U., et al. (2016). How nectar-feeding bats localize their food: echolocation behavior of leptonycteris yerbabuenae approaching cactus flowers. PLoS One 11:e0163492. doi: 10.1371/journal.pone.0163492
Hartley, D. J., and Suthers, R. A. (1987). The sound emission pattern and the acoustical role of the noseleaf in the echolocating bat, Carollia perspicillata. J. Acoust. Soc. Am. 82, 1892–1900. doi: 10.1121/1.395684
Heller, K.-G., and Helversen, O. V. (1989). Resource partitioning of sonar frequency bands in rhinolophoid bats. Oecologia 80, 178–186. doi: 10.1007/BF00380148
Hodgkison, R., Ayasse, M., Häberlein, C., Schulz, S., Zubaid, A., Mustapha, W. A. W., et al. (2013). Fruit bats and bat fruits: the evolution of fruit scent in relation to the foraging behaviour of bats in the New and Old World tropics. Funct. Ecol. 27, 1075–1084. doi: 10.1111/1365-2435.12101
Hoffmann, F. G., Hoofer, S. R., and Baker, R. J. (2008). Molecular dating of the diversification of Phyllostominae bats based on nuclear and mitochondrial DNA sequences. Mol. Phylogen. Evol. 49, 653–658. doi: 10.1016/j.ympev.2008.08.002
Jakobsen, L., Hallam, J., Moss, C. F., and Hedenström, A. (2018). Directionality of nose-emitted echolocation calls from bats without a nose leaf (Plecotus auritus). J. Exp. Biol. 221:jeb.171926. doi: 10.1242/jeb.171926
Jakobsen, L., Ratcliffe, J. M., and Surlykke, A. (2013). Convergent acoustic field of view in echolocating bats. Nature 493, 93–96. doi: 10.1038/nature11664
Jones, G., and Holderied, M. W. (2007). Bat echolocation calls: adaptation and convergent evolution. Proc. Biol. Sci. 274, 905–912. doi: 10.1098/rspb.2006.0200
Jones, P. L., Page, R. A., and Ratcliffe, J. M. (2016). “To scream or to listen? Prey detection and discrimination in animal-eating bats,” in Bat Bioacoustics, Springer Handbook of Auditory Research, eds M. B. Fenton, A. D. Grinnell, A. N. Popper, and R. R. Fay (New York, NY: Springer), 93–116. doi: 10.1007/978-1-4939-3527-7_4
Jung, K., Molinari, J., and Kalko, E. K. V. (2014). Driving factors for the evolution of species-specific echolocation call design in new world free-tailed bats (Molossidae). PLoS One 9:e85279. doi: 10.1371/journal.pone.0085279
Kalko, E. K. V. (2004). Neotropical leaf-nosed bats (Phyllostomidae): “Whispering” bats as candidates for acoustic surveys? Bat. Conserv. Int. 63–69.
Kalko, E. K. V., Villegas, S. E., Schmidt, M., Wegmann, M., and Meyer, C. F. J. (2008). Flying high—assessing the use of the aerosphere by bats. Integr. Comp. Biol. 48, 60–73. doi: 10.1093/icb/icn030
Kalko, E. K., Handley, C. O. Jr., and Handley, D. (1996). Organization, diversity, and long-term dynamics of a Neotropical bat community. Long Term Stud. Verteb. Commun. 503–553. doi: 10.1016/b978-012178075-3/50017-9
Korine, C., and Kalko, E. K. V. (2005). Fruit detection and discrimination by small fruit-eating bats (Phyllostomidae): echolocation call design and olfaction. Behav. Ecol. Sociobiol. 59, 12–23. doi: 10.1007/s00265-005-0003-1
Kounitsky, P., Rydell, J., Amichai, E., Boonman, A., Eitan, O., Weiss, A. J., et al. (2015). Bats adjust their mouth gape to zoom their biosonar field of view. Proc. Natl. Acad. Sci. U.S.A. 112, 6724–6729. doi: 10.1073/pnas.1422843112
Leiser-Miller, L. B., and Santana, S. E. (2020). Morphological diversity in the sensory system of phyllostomid bats: implications for acoustic and dietary ecology. Funct. Ecol. 34, 1416–1427. doi: 10.1111/1365-2435.13561
López-Baucells, A., Rocha, R., Bobrowiec, P. E. D., Palmeirim, J. M., and Meyer, C. F. J. (2016). Field Guide to Amazonian Bats. Manaus: National Institute of Amazonian Research (INPA).
Matsuta, N., Hiryu, S., Fujioka, E., Yamada, Y., Riquimaroux, H., and Watanabe, Y. (2013). Adaptive beam-width control of echolocation sounds by CF-FM bats, Rhinolophus ferrumequinum nippon, during prey-capture flight. J. Exp. Biol. 216, 1210–1218. doi: 10.1242/jeb.081398
Metzner, W., and Müller, R. (2016). “Ultrasound Production, Emission, and Reception,” in Bat Bioacoustic, eds M. Fenton, A. Grinnell, A. Popper, and R. Fay (New York, NY: Springer)
Minin, V. N., Bloomquist, E. W., and Suchard, M. A. (2008). Smooth skyride through a rough skyline: Bayesian coalescent-based inference of population dynamics. Mol. Biol. Evol. 25, 1459–1471. doi: 10.1093/molbev/msn090
Neuweiler, G., Metzner, W., Heilmann, U., Rübsamen, R., Eckrich, M., and Costa, H. H. (1987). Foraging behaviour and echolocation in the rufous horseshoe bat (Rhinolophus rouxi) of Sri Lanka. Behav. Ecol. Sociobiol. 20, 53–67. doi: 10.1007/BF00292166
Pedersen, S. C. (1993). Cephalometric correlates of echolocation in the Chiroptera. J. Morphol. 218, 85–98. doi: 10.1002/jmor.1052180107
Pedersen, S. C. (1995). Cephalometric correlates of echolocation in the Chiroptera: II. Fetal development. J. Morphol. 225, 107–123. doi: 10.1002/jmor.1052250109
Pedersen, S. C. (1998). Morphometric analysis of the chiropteran skull with regard to mode of echolocation. J. Mammal. 79, 91–103. doi: 10.2307/1382844
Ripperger, S. P., Rehse, S., Wacker, S., Kalko, E. K. V., Schulz, S., Rodriguez-Herrera, B., et al. (2019). Nocturnal scent in a “bird-fig”: A cue to attract bats as additional dispersers? PLoS One 14:e0220461. doi: 10.1371/journal.pone.0220461
Rodríguez-San Pedro, A., and Allendes, J. L. (2017). Echolocation calls of free-flying common vampire bats Desmodus rotundus (Chiroptera: phyllostomidae) in Chile. Bioacoustics 26, 153–160. doi: 10.1080/09524622.2016.1231079
Rojas, D., Warsi, O. M., and Dávalos, L. M. (2016). Bats (Chiroptera: Noctilionoidea) challenge a recent origin of extant neotropical diversity. Syst. Biol. 65, 432–448. doi: 10.1093/sysbio/syw011
Rübsamen, R. (1987). Ontogenesis of the echolocation system in the rufous horseshoe bat, Rhinolophus rouxi (Audition and vocalization in early postnatal development). J. Comp. Physiol. 161, 899–913. doi: 10.1007/BF00610231
Schnitzler, H.-U., and Denzinger, A. (2011). Auditory fovea and Doppler shift compensation: adaptations for flutter detection in echolocating bats using CF-FM signals. J. Comp. Physiol. A 197, 541–559. doi: 10.1007/s00359-010-0569-6
Schnitzler, H.-U., and Kalko, E. K. (2001). Echolocation by insect-eating bats. Bioscience 51, 557–569. doi: 10.1641/0006-3568(2001)051[0557:ebieb]2.0.co;2
Seibert, A.-M., Koblitz, J. C., Denzinger, A., and Schnitzler, H.-U. (2015). Bidirectional echolocation in the bat Barbastella barbastellus: different signals of low source level are emitted upward through the nose and downward through the mouth. PLoS One 10:e0135590. doi: 10.1371/journal.pone.0135590
Shapiro, B., Rambaut, A., and Drummond, A. J. (2006). Choosing appropriate substitution models for the phylogenetic analysis of protein-coding sequences. Mol. Biol. Evol. 23, 7–9. doi: 10.1093/molbev/msj021
Stidsholt, L., Greif, S., Goerlitz, H. R., Beedholm, K., Macaulay, J., Johnson, M., et al. (2021). Hunting bats adjust their echolocation to receive weak prey echoes for clutter reduction. Sci. Adv. 7:eabf1367. doi: 10.1126/sciadv.abf1367
Strother, G. K., and Mogus, M. (1970). Acoustical beam patterns for bats: some theoretical considerations. J. Acoust. Soc. Am. 48, 1430–1432. doi: 10.1121/1.1912304
Surlykke, A., Jakobsen, L., Kalko, E. K. V., and Page, R. A. (2013). Echolocation intensity and directionality of perching and flying fringe-lipped bats, Trachops cirrhosus (Phyllostomidae). Front. Physiol. 4:143. doi: 10.3389/fphys.2013.00143
Surlykke, A., Nachtigall, P. E., Fay, R. R., and Popper, A. N. (eds) (2014). Biosonar, Springer Handbook of Auditory Research. New York, NY: Springer New York.
Suthers, R. A., Hartley, D. J., and Wenstrup, J. J. (1988). The acoustic role of tracheal chambers and nasal cavities in the production of sonar pulses by the horseshoe bat, Rhinolophus hildebrandti. J. Comp. Physiol. A 162, 799–813. doi: 10.1007/bf00610969
Taylor, M., and Tuttle, M. (2019). Bats: An Illustrated Guide to All Species. Washington, DC: Smithsonian Books.
Vanderelst, D., De Mey, F., Peremans, H., Geipel, I., Kalko, E., and Firzlaff, U. (2010). What noseleaves do for FM bats depends on their degree of sensorial specialization. PLoS One 5:e11893. doi: 10.1371/journal.pone.0011893
Vanderelst, D., Lee, Y.-F., Geipel, I., Kalko, E. K. V., Kuo, Y.-M., and Peremans, H. (2013). The noseleaf of Rhinolophus formosae focuses the Frequency Modulated (FM) component of the calls. Front. Physiol. 4:191. doi: 10.3389/fphys.2013.00191
Webala, P. W., Rydell, J., Dick, C. W., Musila, S., and Patterson, B. D. (2019). Echolocation calls of high duty-cycle bats (hipposideridae and rhinonycteridae) from Kenya. J. Bat Res. Conserv. 12, 10–20
Weinbeer, M., and Kalko, E. K. V. (2007). Ecological niche and phylogeny: the highly complex echolocation behavior of the trawling long-legged bat, Macrophyllum macrophyllum. Behav. Ecol. Sociobiol. 61, 1337–1348. doi: 10.1007/s00265-007-0364-8
Wilson, D. E., and Mittermeier, R. A. (eds) (2019). Handbook of the Mammals of the World, 1st ed, Handbook of Mammals of the World. Barcelona: Lynx Edicions.
Yoh, N., Syme, P., Rocha, R., Meyer, C. F. J., and López-Baucells, A. (2020). Echolocation of Central Amazonian ‘whispering’ phyllostomid bats: call design and interspecific variation. Mamm. Res. 65, 583–597. doi: 10.1007/s13364-020-00503-0
Keywords: behavior, evolution, echolocation, oral emission, nasal emission, noseleaf, Phyllostomidae
Citation: Gessinger G, Page R, Wilfert L, Surlykke A, Brinkløv S and Tschapka M (2021) Phylogenetic Patterns in Mouth Posture and Echolocation Emission Behavior of Phyllostomid Bats. Front. Ecol. Evol. 9:630481. doi: 10.3389/fevo.2021.630481
Received: 17 November 2020; Accepted: 15 April 2021;
Published: 10 May 2021.
Edited by:
Brock Fenton, Western University (Canada), CanadaReviewed by:
James A. Simmons, Brown University, United StatesSharlene Santana, University of Washington, United States
Elizabeth Dumont, University of California, Merced, United States
Copyright © 2021 Gessinger, Page, Wilfert, Surlykke, Brinkløv and Tschapka. This is an open-access article distributed under the terms of the Creative Commons Attribution License (CC BY). The use, distribution or reproduction in other forums is permitted, provided the original author(s) and the copyright owner(s) are credited and that the original publication in this journal is cited, in accordance with accepted academic practice. No use, distribution or reproduction is permitted which does not comply with these terms.
*Correspondence: Gloria Gessinger, gloria.gessinger@alumni.uni-ulm.de